The European Union (EU)’s goal is to have 10 million electric vehicles (EVs) sold in the EU by 2030. In order to hit this target, the number of EVs sold in the EU needs to ramp up drastically. In its aspirations to reach this target, Europe emphasizes securing its independence as a producer of the lithium-ion batteries (LiBs) that power EVs through the construction of so-called battery gigafactories. Though gigafactories are absolutely required to supply the most important part of a battery electric vehicle (BEV), the manufacturing of battery cells is just one link in the battery manufacturing chain. Securing the right set of battery minerals upstream and turning them into battery chemistries that meet the quality requirements of original equipment manufacturers (OEMs) is of equal, if not more, importance. This article describes the impact that battery minerals have on the production costs of LiBs, how it echoes through the entire LiB supply chain and, eventually, affects the EU’s chances to create a mass market for BEVs on European soil.
The drivers behind EV costs
Around 36% of the cost of producing a BEV relates to producing the car’s LiB cell and pack (Figure 2). The US Department of Energy believes that LiB prices need to drop below US$100/kWh for BEVs to reach price parity with internal combustion engine (ICE) cars. As illustrated in the Bloomberg New Energy Finance (BNEF) graph (Figure 3), the price of LiBs has come down significantly. Earlier this year, BNEF announced that a set of LiBs was sold even below US$100/kwh. If OEMs like Tesla succeed in offering BEVs at a price of US$25,000, the chances of creating a mass market for BEVs will improve significantly.
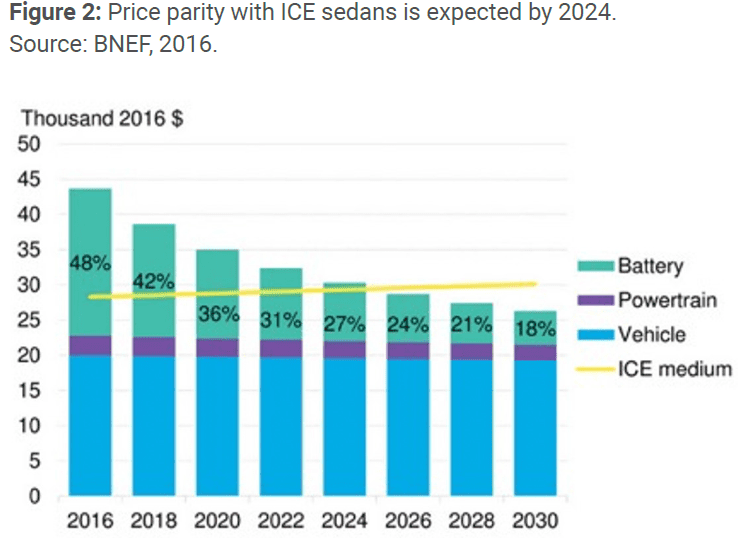
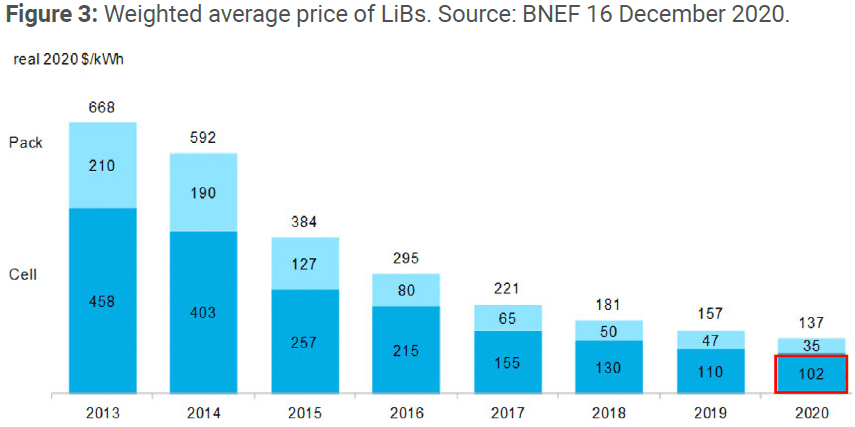
The most commonly used battery minerals are lithium, nickel, cobalt, manganese, and aluminium for cathodes and graphite for anodes. Minerals need to be chemically processed before being useful in end-applications like BEVs. The OEMs dictate the quality requirements for LiBs that go into their BEVs. Different battery chemistries react differently when charged and discharged multiple times and it’s extremely important for OEMs to find battery chemistries that strike the right balance between safety, range, longevity, and price to comfort the consumer. High performing, reliable batteries require cathode materials that have high purity levels and are almost entirely free of metal impurities. Metal impurities cause damage to the LiB, which eventually may compromise the safety of the user.
Gigafactories alone are not sufficient to set up your own regionalized supply chain. Mega investments in the mining, processing, and chemical engineering of battery minerals are required to produce LiBs with the highest standard to provide cars for BEV buyers that are safe, affordable, and have a practical range. Only then will BEVs be able to truly compete with ICE vehicles.
Different cathodes, different materials
Since the LiB cell constitutes approximately 80% of an LiB’s production costs, understanding what contributes to the cost of making an LiB cell is key. When looking at Figure 4, three things become clear. First, cathode Bill of Materials (BoM) costs could easily double if prices of battery minerals were to return to decade highs. Second, the overall costs related to cathodes is large; more than 50% of the LiB cell’s cost is related to making the cathode. Third, cathode and anode together constitute 65-75% of total LiB cell costs. This last point illustrates the importance of the battery minerals that constitute the anode and cathode BoMs as well as the chemical processes required to upgrade these minerals to high purity so that they can be used in LiBs. Finally, it is important to note that this cost breakdown is made on the assumption of the BoM for a NMC 811 cell. This is a high nickel and low cobalt-content cell. Different cathodes have different battery minerals and therefore different BoMs. Exposure to a supply sensitive battery mineral like cobalt can be reduced by using cobalt-free cathodes, such as lithium iron phosphate (LFP) cathodes. But cobalt is not the only mineral with supply issues. Nickel, manganese and lithium can all become supply sensitive minerals too, unless private and public LiB supply chains start expanding the scope of their investments into upstream sections, too.
How does this impact Europe’s EV ambitions?
The next price hike could stagnate the downward trend of LiB prices. In the two scenarios illustrated in Figure 4, the total LiB cell cost increases by almost 36.5% to US$119/kWh. Assuming that an average BEV in Europe will contain a 100kWh energy unit, a 36.5% increase of a NMC 811 LiB cell price could easily lead to a US$3,000 price increase for a BEV. This does not yet include the margin that the BEV OEM will want to make on the car. And we have to bear in mind that possible price hikes for manganese have been left out. Table 1 below indicates the percentage increase in raw materials used in Volkswagen’s EVs from 2018 to 2020. Within the next decade, Volkswagen alone intends to increase its LiB output almost tenfold to 240 gigawatt hours (GWh) in Europe alone. All the major OEMs have announced similar ambitions within the next decade, which can lead to only one thing: an upward pressure on the prices of battery minerals.
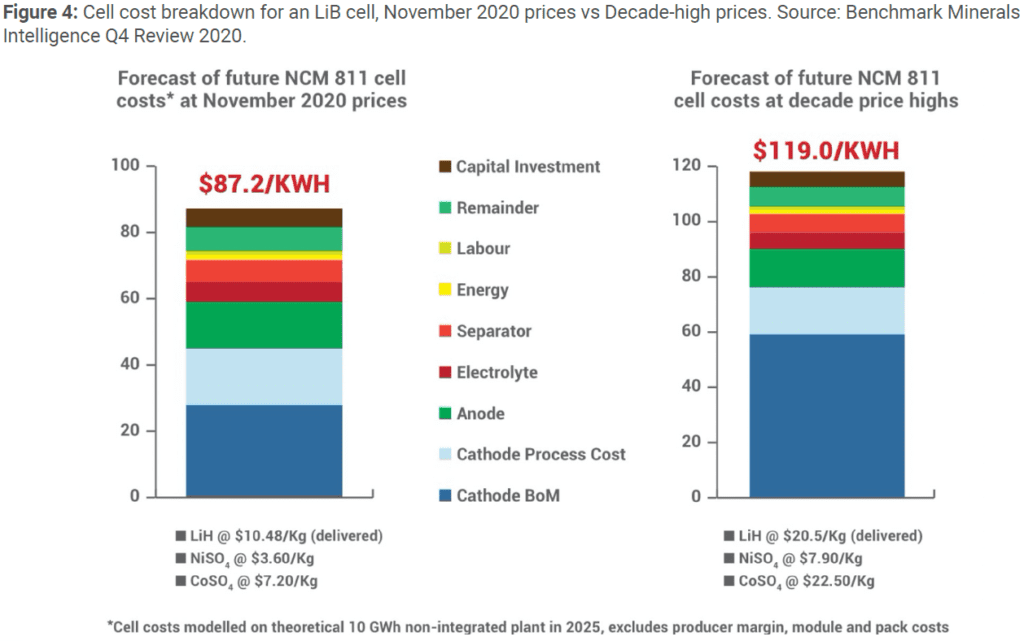
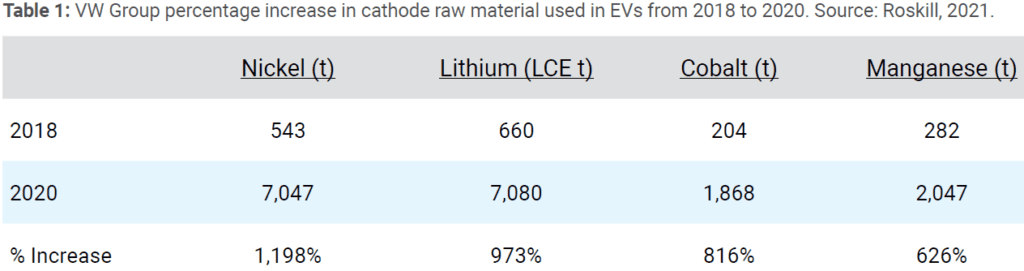
How can policymakers mitigate the price risks related to battery minerals?
Diversification of investments
Figure 5 illustrates the issue well. The larger part of investments in green tech is flowing to projects downstream (i.e. EV and gigafactory roll out). In Europe, most of the attention is going to the gigafactories. With the recent announcement by Volkswagen that it will increase its LiB production in Europe to 240 GWh, Europe’s LiB production capacity is set to become almost 750 GWh. No European OEM will, however, invest in projects upstream (i.e. mining) or midstream (refining and processing). The gap between cumulative downstream investments and cumulative investments upstream over the past three years is a whopping US$80 billion. Depending on the size of the energy unit in these 10 million EVs, Europe will require a multiple of today’s entire global production of lithium compounds (roughly 360,000 tonnes). Since it takes at least seven years to get the average mine into commercial production – and the EU is renowned for its long permitting and stakeholder participation procedures – it looks unlikely that Europe will meet its demand for minerals from local production alone.
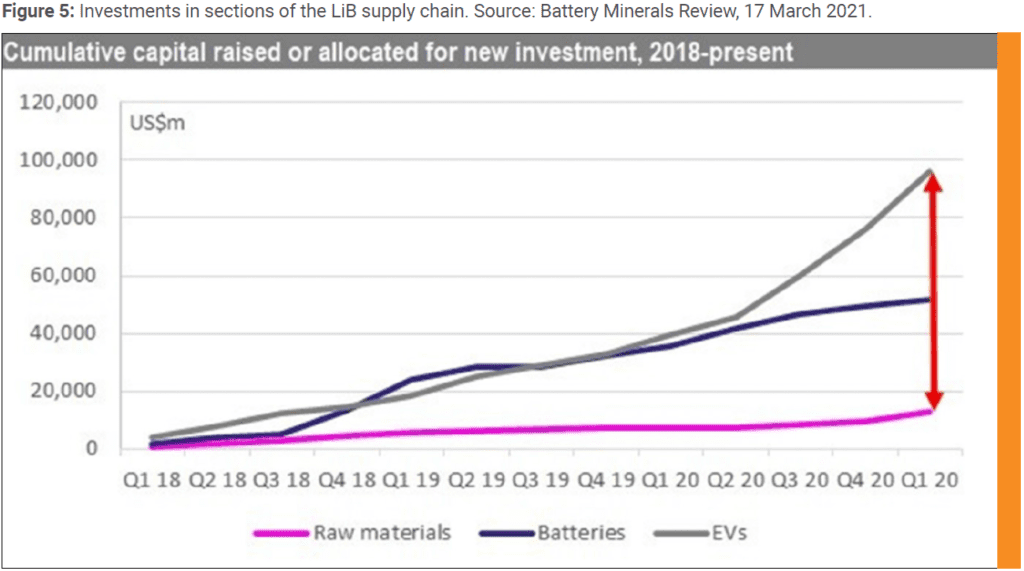
The message that Figures 4 and 5 convey is clear: more funds need to be allocated to the upstream sections in order to secure a steady supply of minerals.
Diversification of materials
The EU has the power to encourage or discourage the use of certain materials by producers in the LiB supply chain. Demanding environmental, social and corporate governance (ESG) certifications from producers to show where their cobalt comes from is a good example. This is not to say that cobalt will disappear entirely as a cathode active material. On the contrary, cobalt still has a role to play, given its benevolent properties as a stabilizer in LiBs.
However, over the next decade there will be a new equilibrium in which the balance of power will shift in favour of high nickel, high manganese and low cobalt chemistries for batteries. Diversification of materials also means looking beyond current LiB technology.
Diversification of sources
Using more nickel and reducing cobalt in LiB cells doesn’t mean that, all of a sudden, ESG standards are met and all producers and suppliers in the LiB supply chain are in compliance with the EU’s ESG requirements. Nickel, too, has its challenges. Nickel sulphates (a speciality chemical used in cathodes) require intermediate nickel products such as nickel matte. By the end of this decade, 40% of these intermediates will be produced and processed in Indonesia, Russia, and China. Ever since Indonesia announced an export ban of nickel ore, the West has become aware that it is not only China and Russia that pose supply risks. Instead of pursuing its legal battles with Indonesia at the World Trade Organisation (WTO), the EU could perhaps consider an approach similar to Chinese and Japanese firms who bring the much needed jobs and funding into the country. Similar risks apply to manganese. High-purity manganese sulphate is required for cathode active materials (CAM) and a shift to high nickel or high manganese-content cathodes will create an upward pressure in the demand for high-purity manganese, a mineral that is not even listed on the EU’s list of critical minerals. This situation is quite difficult to understand given that high-purity manganese sulphate has a limited feedstock at the moment. Today, it is only economically viable to extract high-purity manganese from ores containing manganese carbonate. Only 2% of all manganese-holding orebodies in the world are manganese carbonate ores.
Diversification of materials also means looking beyond current LiB technology
Diversification of products
Market differentiation is not a new phenomenon. BEV OEMs adopt their marketing to meet the needs and purchasing power of consumers in each geography, and even each demographic group, in the most effective way. It is no coincidence that Tesla’s first product in China was the Tesla Model 3, the least expensive model in the Tesla product portfolio. In a country where the central government is actively pushing the rollout of EV infrastructure, range anxiety becomes less of an issue and people can be comfortable with LFP powered BEVs even though they have a smaller range than LiBs with high nickel content cathode chemistries.
The more public charging stations, the quicker range anxiety will disappear as a concern for consumers and the more diverse the portfolio of LiB cathode chemistries can become. Driving electric will not be for the happy few who can afford a BEV with high-performing, premium LiBs; there will be a real mass market for BEVs with a diverse set of products OEMs can offer to different types of consumers with different needs and spending power. This diversification contributes to reducing the exposure to high nickel and high cobalt cathodes, making BEVs less sensitive to large fluctuations in battery mineral prices. With the release of the IP for LFP technology in 2022, I expect that more battery producers in the EU will invest in improving LFP technology which will greatly benefit the European consumer as it will offer a wider range of quality products to choose from.
Conclusion
Battery minerals price hikes adversely affect the price of LiBs. Since LiBs constitute almost 40% of a BEV’s production costs, price hikes upstream steer BEVs away from price parity with ICE vehicles, an important incentive for consumers to switch from ICE vehicles to BEVs. All signs indicate that the market for battery minerals is tightening up. The EU should therefore endeavour to engage more actively in the global LiB supply chain, from upstream to downstream, to make sure it comes close to achieving the targets it has set for itself. Batteries require battery quality minerals so you better make sure you have a foot (or two) in all sections of the LiB supply chain. Not just the fancy ones.