The anatomy of an EV battery
Electric vehicles (EVs) have been front and centre in the past few years, disrupting a traditionally internal combustion engine market. Most EVs run on lithium-ion (li-ion) batteries, the same type of battery used in e-bikes, laptops, and smartphones.
According to McKinsey & Co, growing EV use is expected to increase lithium production by approximately 20% per year this decade, and by 2030, EVs will account for 95% of lithium demand.
While the base component is self-explanatory and does require lithium, the rest of an EVs battery make up varies from company to company, and between car models. The makeup of these li-ion batteries can affect an EVs energy density (vehicle range), charge time, and safety (flammability).
A slight shift in chemistry can change these variables, with all mixes exhibiting different performance characteristics. For instance, lithium-nickel-manganese-cobalt-oxide (NMC) batteries have high energy density due to the cobalt content of its cathode, which supports better driving distance range.
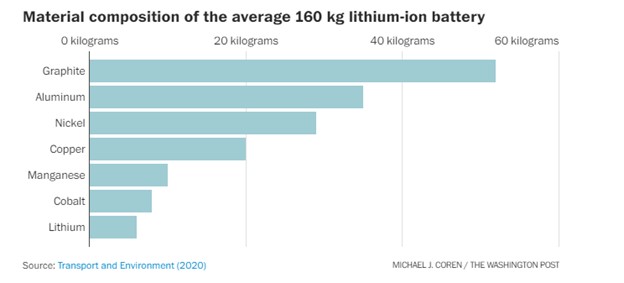
EV batteries function by circulating electrons between two electrodes, creating a potential difference. One electrode, known as the anode, carries a negative charge, while the other electrode, the cathode, holds a positive charge. These electrodes are submerged in a conductive liquid called the electrolyte.
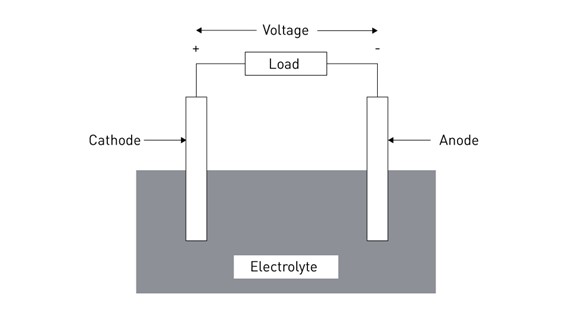
When the battery powers the vehicle, electrons flow from the anode to the cathode. Conversely, during charging, the electron flow is reversed, moving from the cathode to the anode. The basic elements of a battery cell are shown in the image above.
Anodes are typically made from graphite, whereas the electrolyte is a liquid or gel lithium salt. The cathode is made from lithium metal oxide combinations of cobalt, nickel, manganese, iron, and aluminium, and its composition largely determines battery performance.
The EV market is poised for rapid growth, and the surge in demand presents both opportunities and challenges for the lithium industry.
To meet burgeoning demand, more lithium mines are coming online. However, according to estimates from Rystad Energy, this increased supply will only provide a temporary solution, extending the availability of lithium resources for only a few more years.
The lithium sector is currently witnessing an active exploration phase, with hundreds of projects under exploration. However, the industry faces significant hurdles due to the complexity of geological conditions and the time-consuming permitting processes. Despite the ongoing lithium exploration efforts, the challenges persist.
Refinitiv data reveals that there are currently only 101 lithium mines worldwide, highlighting the limited availability of this critical resource. As EV sales surge and the demand for lithium intensifies, the industry must grapple with meeting this growing appetite.
Battery applications
Batteries play a crucial function in the contemporary world. The technology we use and the way we live have changed dramatically because of battery mobility and energy storage capabilities. Batteries have diverse applications that have transformed many sectors.
In the realm of mobility and communication, batteries power smartwatches, tablets, cell phones, and laptops, enabling seamless communication and information access. In the medical field, batteries are essential for devices like hearing aids, pacemakers, and portable medical equipment, providing flexibility and independence in patient care.
During emergencies and power outages, batteries serve as crucial energy sources for radios, flashlights, and other life-saving supplies. Additionally, batteries power consumer electronics such as remote controls, cameras, and toys, making them portable and convenient.
Moreover, batteries play a vital role in renewable energy storage, enhancing the reliability and effectiveness of solar and wind power systems.
Finally, batteries are at the forefront of sustainable transportation with the rise of electric cars, reducing pollution and greenhouse gas emissions by eliminating the need for fossil fuels.
What’s the status of competing battery technologies right now?
Until recently, most li-ion batteries were available in either lithium-iron-phosphate (LFP, low-energy density but high safety) or NMC (high performance) chemistries but today the range of battery products is far more diverse. Compared to only five years ago, the chemistries have greatly changed as issues around EV range, safety, and overall costs drive further innovation.
Replacing some iron content with manganese in LFP cells, or LMFP batteries, has reduced the energy density gap in NMC chemistries. For the nickel-based chemistries, cobalt is being phased out and even eliminated to the benefit of nickel content due to both environmental damage and social concerns.
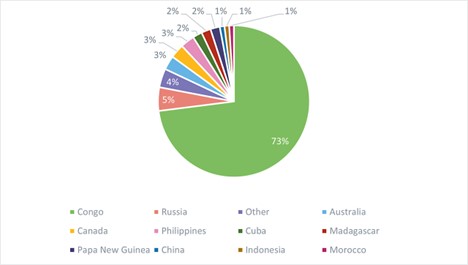
With lithium-based chemistries maturing, the current hype has drawn eyes towards other chemistries that will be explored further in the next section.
Battery compositions comparison
The future of the battery industry will depend on its ability to continue delivering breakthrough battery technology and alternative chemistries if net-zero goals are to be achieved.
There are alternative battery chemistries emerging, yet it’s important to note that there is not an alternative to li-ion batteries with equal energy density, which can be rolled out commercially. Energy density determines the EV’s range – so this will be a crucial challenge to overcome if we are to see alternative batteries take dominant positions in the global market.
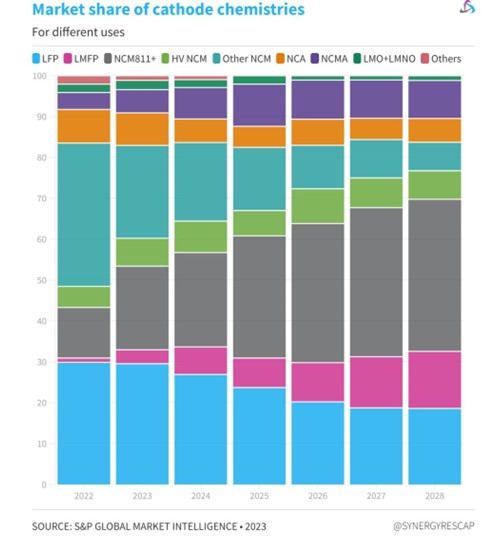
Below are the most notable alternatives:
- Lithium-iron-phosphate (LFP): LFP batteries are becoming popular in EVs from European manufacturers. They contain no cobalt, instead using iron and phosphate, which are cheaper, more abundant materials in the earth. The batteries have less energy density, but better thermal safety than a typical li-ion battery. Tesla now uses LFP batteries in its standard Model 3 and Model Y cars, which have lower range requirements.
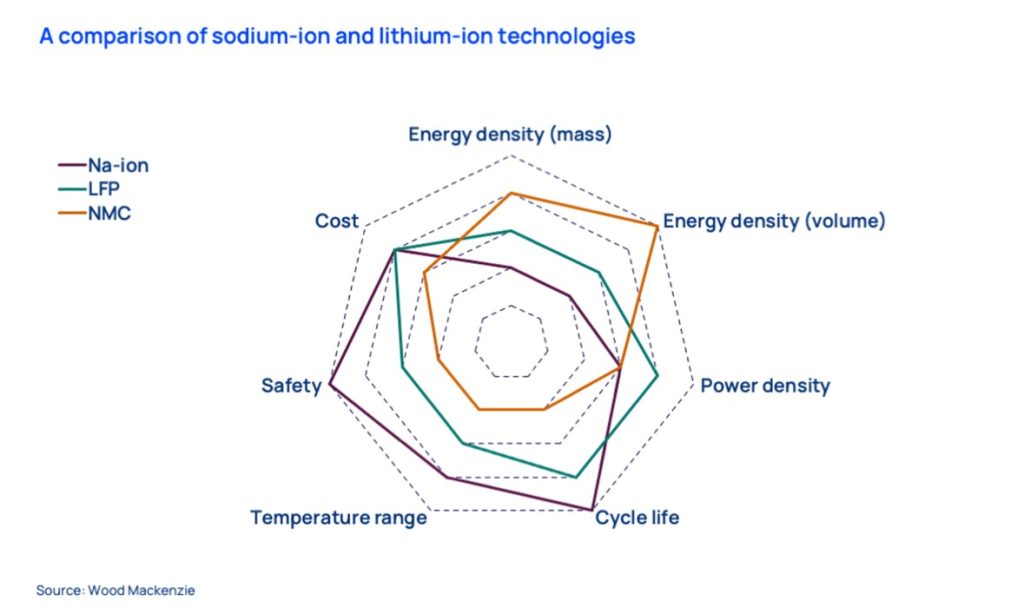
- Sodium-ion (SIB): This early-stage technology is not yet commercialized. It uses aluminium and sodium, which is more than 1,000 times as naturally abundant as lithium. However, SIB batteries have less energy density/vehicle range than average li-ion batteries, and are heavier, making them more suitable for energy storage perhaps in places like the US where drivers value longer driving ranges.
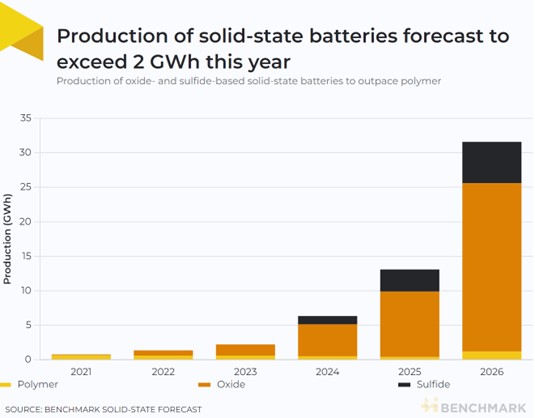
- Solid-State: These batteries use a solid electrolyte as opposed to the liquid gel found in current li-ion batteries. These batteries do contain cobalt, but in much lower amounts than today’s batteries, and offer advantages for power density and a much lower risk of fire. Many manufacturers, including Toyota, Samsung and LGES, are aiming to reach commercialization for solid-state batteries over the next few years. This swap unlocks possibilities that pack more energy into a smaller space, potentially improving the range of EVs. Solid-state batteries could also have shorter charging times, making them an attractive newer technology.
Improvements in battery technology can be achieved in a huge range of different ways and focus on several different components to deliver certain performance characteristics of the battery. While there are various paths that battery technology evolution could take, S&P Global has defined three new alternatives to lithium-ion batteries.
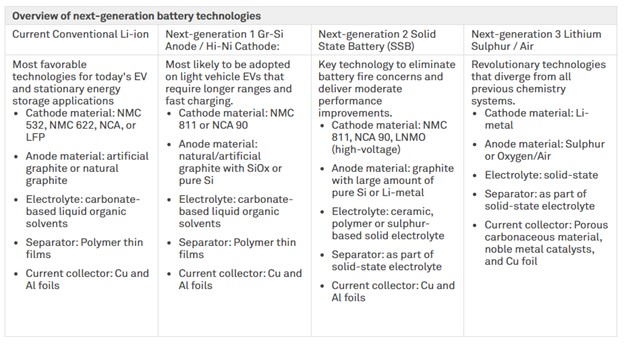
It’s clear that innovation in battery technologies is necessary to be in with a fighting chance of achieving net-zero. There is no one-size-fits-all solution, with vastly different demands to electrify the transport sector alone. Using different technologies is the optimal method to meet the varying demands from the many applications that require batteries.
What challenges remain?
These other chemistries will become more competitive in the coming years, they are not yet full contenders against li-ion batteries as is. For now, the best approach is still to bridge the gap in looming supply deficits for many battery metals through mineral extraction.
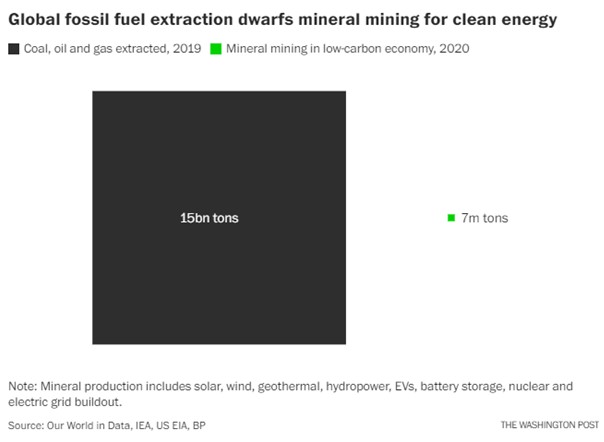
To compare EVs with conventional ICE vehicles, it’s only natural to compare the extractions necessary for each and what it takes to fuel them. Mining is typically measured in millions of tonnes per year. For fossil fuel extraction, that’s a rounding error.
In 2020, building the world’s wind turbines, solar panels, EVs, and other clean-energy infrastructure demanded 7Mt of minerals, according to the International Energy Agency. Roughly half of this was destined for batteries and EVs.
Clean-energy technologies have the potential to utilize these materials for decades, and if properly recycled, their lifespan can extend indefinitely. The longevity and sustainability offered by clean-energy technology present a compelling argument for a transition towards a greener future, making the future for EVs and their upcoming battery innovations even more necessary and groundbreaking.